Engineered Cell Therapies
By Niko McCarty
Cell Therapy
In 2010, two patients with chronic lymphocytic leukemia, a form of blood cancer, received an experimental therapy.
T-cells, a type of white blood cell that helps the body to mount an immune response, were carefully extracted from each person’s blood. The T-cells were then engineered to express a protein known as a CAR, which stands for chimeric antigen receptor. To accomplish this, a gene expression cassette for the CAR protein was packaged as RNA into a lentiviral vector (a type of engineered HIV that can’t replicate, and where the HIV genome is replaced with a synthetic genetic payload, in this case the CAR), and then mixed with the T-cells. The lentiviruses entered the patients’ T-cells and delivered the payload-containing RNA molecules, which were then reverse transcribed into DNA by lentiviral enzymes and integrated into the recipient cells’ genomes.
After several days, the T-cells began to make the CAR protein. These particular CAR proteins were engineered to recognize a specific antigen associated with leukemia cells, converting that into a signal that the T-cell should attack. The T-cells, thus re-wired, were placed back into the bodies from which they came. In this way, the patient's immune system had effectively been taught how to eradicate cancer cells as though they were any other intruder.
Carl June, the oncologist in charge of the therapy, was not initially optimistic that the therapy would work long-term, however. He told reporters that he expected that the CAR-T cells “would be gone in a month or two,” and the cancers would likely persist or come back. But that’s not what happened.
Both patients had complete remission. Their cancer melted away. And after more than a decade, things remained that way. The engineered T-cells lived on in the body and continued to fight back against rogue cancer cells. (One of the two patients, unfortunately, recently died from COVID-19.)
June’s study was an early demonstration of the power of engineered cell therapy. It suggested, for the first time, that engineered immune cells could hunt down and destroy cancer. And it set off a blitz to search for other forms of cancer — or other diseases entirely — to go after.
The Food and Drug Administration (F.D.A.) has now approved dozens of cell therapy products, nearly half of which are engineered, meaning that the cells are altered to carry added transgenes — a CAR, a toll-like receptor, or something else. Engineered cell therapies are any clinical intervention in which modified cells are placed into the body to correct a disease or replace existing tissues. CAR-T therapy is probably the most notable example, but there are other applications, too.
At least 3,900 cell, gene, and RNA therapies are in clinical or preclinical development, according to a 2023 report from the American Society of Gene + Cell Therapy.
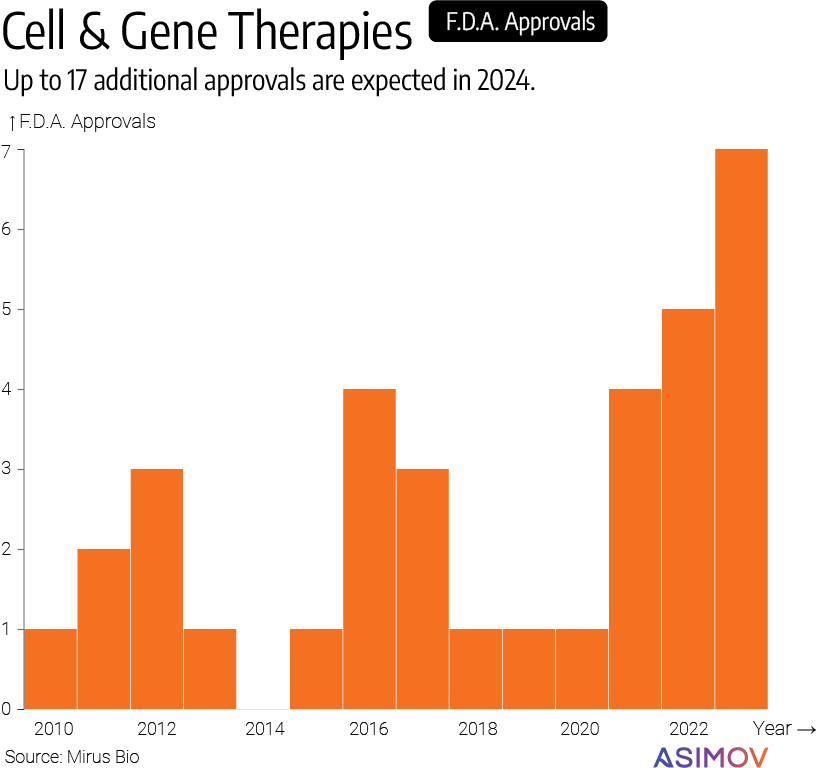
Lyfgenia, for example, is a cell therapy that is used to treat sickle-cell disease. One of the terrible things about this particular disease is the pain it inflicts upon a person. The condition impairs blood cells from carrying enough oxygen through the body, due to a genetic mutation that causes abnormal hemoglobin protein. It also makes those cells become misshapen and ‘sticky,’ such that they begin to clump together. The sickle-shaped cells can block vessels and cause shooting pains or, in some cases, death.
The way that Lyfgenia works is relatively straightforward: Scientists collect the stem cells that make blood from a patient’s body, and then genetically alter them so that they make a functional form of the hemoglobin protein. The engineered stem cells are then reinfused back into the body, and begin to make healthy blood cells. In a clinical trial of 47 people who received Lyfgenia, the episodes of searing pain “were eliminated or significantly reduced in all patients.”
Apologies for the editorializing, but that’s incredible.
Despite the many cell therapies that are now coming onto the market, CAR-T therapies remain the most popular. There is, for example, an FDA-approved CAR-T therapy that can induce complete cancer remission in up to 90 percent of patients who have relapsed or refractory B-cell acute lymphoblastic leukemia, or B-ALL.
Physicians who would once hesitate to use the word “cure” for cancer treatments are now lobbing it about in press releases. Over the next two decades, millions of people will likely receive cell therapy. That’s a great thing. But it will also come with its fair share of challenges.
Among them: How do we safely, reliably, and efficiently get DNA into a patient's cells? You can't just inject DNA into a patient, or even into a cell, so we need another way. Viruses are the natural solution to this problem, but they come with their own challenges, too.
Many cell therapies today are made by using lentiviruses, which enter the patient’s cells and act as a sort of vessel that endows them with new genetic material. Currently, this step is performed outside the body and the engineered cells are infused back into the patient. In the future, this delivery vessel may be able to be injected straight into the bloodstream.
Lentiviruses are an entire genus of viruses that infect humans, cows, pigs, and other mammals. The lentivirus used to make cell therapies came from HIV, and was tested in a clinical trial for the first time in 2003, with a total of 65 patients. In that trial, the lentivirus was used to introduce a ‘genetic payload’ into T-cells to treat — of all things — an HIV infection. None of the patients had an adverse event as a result of the lentivirus, even after 8 years of monitoring.
And then, in 2017, Kymriah became the first F.D.A.-approved cell therapy made using a lentivirus. Kymriah is a treatment for B-ALL and non-Hodgkin lymphoma. In the next few years, many more lentivirus-made cell therapies will get F.D.A. approval. Current cell therapies are typically autologous, which means they use a patient’s own cells to fix a disease. This means that the entire supply chain is effectively patient-specific.
Demand for lentiviral vectors is expected to grow in the next few years, according to McKinsey & Co., especially as dozens of lentivirus-made cell therapies that are currently in clinical trials could soon reach the market. When that happens, “manufacturers could struggle to meet demand,” according to a recent report by Endpoints News.
It’s one thing to manufacture a small number of cell therapies for participants in a clinical trial, but a different beast entirely to scale up manufacturing for tens of thousands of patients. We need to make lentivirus manufacturing both more affordable and easier to scale if we ever want engineered cell therapies to help millions of people.
Bring the Payload
The term “lentivirus” derives from the Greek word for lenti-, meaning “slow.” These viruses were first discovered circulating as “a slowly progressive disorder” amongst sheep flocks in Iceland. A long period of time, ranging from several months to years, often elapses between the initial infection of lentivirus and the onset of a disease. But although lentiviruses are ancient — at least 7 million years old — most of the progress in adapting them to cure diseases has happened in the last two decades.
Each lentivirus carries strands of RNA that are reverse transcribed into DNA and then integrated into a host’s genome after infection. This feature is precisely why lentiviruses were adopted for cell therapies in the first place: Their genetic payloads weasel their way into the host genome and thus pass on “stable” copies of genetic information. If a scientist uses a lentivirus to engineer a stem cell, for example, then that stem cell will continue to divide into otherwise normal cells carrying the transgene.
Another advantage of using lentiviruses, as opposed to adeno-associated viruses, or AAVs, is that they are much larger. A typical lentivirus can carry about 10,000 nucleotides, more than twice as much as AAV, which is useful when the payload you want to deliver is a larger gene.
Still, lentiviruses have their own problems. For one, they tend to integrate genetic material in a semi-random manner, which means there is always a chance — however slight — that a lentivirus will insert its ‘payload’ into an important part of the cell’s genome, and cause cancer. The risk is not zero. AAVs, for context, are typically non-integrating, which means their genetic material payloads don’t get stably passed on to a cell’s progeny.
By December 2023, the F.D.A. had received reports of at least “22 cases of T-cell cancers that occurred after treatment with CAR-T products,” according to a recent article in The New England Journal of Medicine. The agency has introduced additional monitoring requirements as a result, but it’s not clear exactly what causes these cancers.
Lentiviruses are also expensive to make. A typical CAR-T therapy costs anywhere from $375,000 to $1,000,000, a price that is being paid by some insurance companies in the U.S., but that is causing European health systems to balk. Part of this high cost stems from the ways in which a lentivirus is made in the laboratory.
Four plasmids, or loops of DNA, are required to make a lentivirus. Each of these plasmids carries genes that encode a different part of the virus. The env plasmid encodes glycoproteins, which sit in the virus’ outer envelope and help it target certain cells. The gag-pol and rev plasmids encode retrovirus structural proteins and things like reverse transcriptase, the enzyme required for the virus to convert its RNA payload into DNA before integration into the genome. The fourth plasmid encodes the gene of interest; a CAR or something else. Lentiviruses are made with four individual plasmids to reduce the risk that a lentivirus, once created, can continue to reproduce on its own and cause disease. Splitting up the plasmids makes the viruses safer; it deactivates them.
All of these plasmids are wrapped up in a polymer bubble and then transfected, or delivered across the cell membrane and into the nucleus of production cells. Most companies use HEK293 or its derivatives to make lentiviruses. HEK293 cells were made in 1973 by culturing kidney cells from a human embryo. When lentivirus plasmids are added to HEK293 cells, they begin to make the viruses. And that’s the basic gist of lentivirus manufacturing.
But here’s the problem: The plasmids themselves are a ‘reagent’ in the manufacturing. In order for the lentivirus to be used in an F.D.A.-approved therapy, the plasmids must be certified as GMP-grade, meaning they are safe, pure, effective, and traceable. GMP-grade plasmids are inordinately expensive, costing in the neighborhood of hundreds of thousands of dollars per batch. It also takes between six months to a year, after ordering, to receive GMP-grade plasmids. This is a major bottleneck in lentivirus manufacturing.
Lentivirus manufacturing is typically done in 200 liter or 1,000 liter bioreactors. Each bioreactor run costs somewhere in the mid-seven figure range, and has to be carefully managed to ensure that there is relative consistency between one run and the next.
All of these challenges make it difficult for companies, especially startups, to compete in this space. There are not enough companies making GMP-grade plasmids, and the scalability of the production process isn't good enough to meet future demand for cell therapies.. We need to reduce costs, improve consistency, and boost scales.
LV Edge
Between 2017 and 2023, at least 20,000 people received CAR-T therapy. But now, CAR-Ts are being engineered to target an even wider range of cancers. To keep costs in check and reach a wider number of people, lentivirus production will need to scale accordingly.
All of the current F.D.A.-approved cancer cell therapies go after blood-based cancers. Carvykti is used to treat multiple myeloma, Kymriah is used to treat B-cell lymphomas, and so on. Mechanistically, Kymriah works by reprogramming a person’s T-cells to express a CD19-specific CAR. This CAR protein is embedded in the T-cell membrane and sticks out like a thumb. When the CAR protein binds to a cancer cell or a B-cell with a specific antigen, the T-cell becomes “activated” and mounts an immune response. The cancer cells are killed, cytokines are released, macrophages are activated.
It is more difficult to make CAR-T therapies that target solid tumors, though, for a few reasons. Among them: Many of the proteins that a CAR recognizes, on the surface of a cancer cell, also show up at a low level in healthy cells. That makes it challenging to make a CAR-T therapy against solid tumors that kills cancer cells but leaves everything else unscathed. Solid tumors also shroud themselves in an acidic environment that helps to keep out non-cancer cells, and diminishes the ability of T-cells to differentiate and mount an immune response.
But this is changing, and many more engineered CAR-T therapies are expected to hit the market soon. An early-stage clinical trial in 16 patients who had a solid tumor expressing a specific antigen, called CLDN6, found that CAR-T treatment led to a partial remission of testicular or ovarian cancer in six of them. There are dozens of other ongoing clinical trials for CAR-T therapies in solid tumors (including some in which macrophages, another type of white blood cell, are engineered to express CARs), for everything from lung to brain to liver cancers.
It would be great if these trials worked out. We really hope they do. CAR-T therapy is akin to engineering the immune system to recognize and destroy the cancer cells that it had failed to detect. This will help millions of people, but only if we’re ready to meet the demands. LV Edge can help with some of these problems.
As a start, we’re rolling out a line of HEK293 cells in which the important genes needed to make lentivirus (env, gag-pol, and rev) are stably integrated into the genome. Companies who use our platform will receive access to this cell line, which could save them hundreds of thousands of dollars in GMP-grade plasmids per batch. One only needs to transiently transfect the HEK293 cells with a plasmid encoding the gene of interest (a particular CAR, say), and then induce, or “switch on,” expression of the lentivirus-making machinery. We call this our “Packaging” cell line.
We’ll soon roll out a “Producer” cell line, too, that has all of the lentivirus-making genes — including the gene of interest — stably integrated in the genome, without impacting titer. LV Edge could drastically reduce costs for companies seeking to move into the cell therapy space. Our cell lines routinely achieve titers between 1E8 and 1E9 TU/ml (transducing units per milliliter, where a single TU corresponds to one integration event in a target cell). Our cell lines also maintain >90% stability for dozens of generations.
Our LV Edge system includes a suite of computational tools to optimize the expression of your CAR (or any other gene of interest, for that matter) and ready-to-transfer processes and protocols for companies to scale up lentivirus production. We’ll share data on all of this, and more, in part II.
Join us or subscribe below for updates.
Contributors: Jamie Freeman, Raja Srinivas, Martín Cárcamo, Jeff McMahan, Brendan Eder, Chris Thorne, Jeremy Gam, Ben Gordon, Alec Nielsen. Words by Niko McCarty.
Image Credit: The header image is a scanning electron micrograph of a human T lymphocyte, or T-cell, from a healthy person. Credit: NIAID